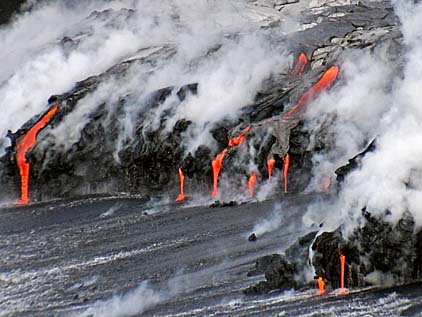
STAR-BULLETIN
The minerals in volcanic rocks form at high temperatures. After cooling, they no longer are in their equilibrium conditions and are unstable. Through weathering, the rocks' atoms rearrange themselves to form new minerals, clay and iron oxides. These are stable at surface temperatures and form the bulk of our soil in Hawaii.
|
|
Natural systems constantly
aim for equilibrium
When we hear the word equilibrium we think of balance, and one of the dictionary meanings refers to the ability of we bipeds to maintain our balance while walking upright, which is definitely a type of balance.
But another type of balance, and a deeper meaning of the word refers to one of the most important, yet easily overlooked principles that pervades virtually all fields of human inquiry, not only in the hard physical sciences such as physics, chemistry, geology and meteorology, but also in biology and the social sciences such as economics, psychology and history.
It seem such a simple concept, not unlike the balance used to weigh things ... or on a see-saw.
When the see-saw is balanced we can say that the two unequal weights have reached a stable equilibrium.
This way of visualizing equilibrium is one that is common in that branch of engineering known as statics. When civil engineers are considering the forces that will be exerted on a building or a bridge they want to be sure that the structure is strong enough to maintain the balance of forces against whatever load will be applied, thus maintaining the equilibrium state.
A state of equilibrium, whether stable or unstable does not mean that there are no forces or other agents of change acting, but only that they are balanced. If there are no changes in the influencing factors the system is said to be in a state of static equilibrium.
In nature, much of the equilibrium is dynamic. Dynamic equilibrium is a state where change is occurring, but in such a way as to maintain the balance.
Thermodynamic equilibrium, for example, is achieved when two objects are placed in contact and heat flows from one to the other until they eventually reach the same temperature. This does not mean that there is no longer any interaction between them, it merely means that each loses and gains the same amount of heat as the other, keeping the temperature constant in both.
In general, nature tends toward a state of dynamic equilibrium but never gets there because nature is never static.
As an everyday example of dynamic equilibrium, consider a bathtub that is filled to a certain level, then the plug is removed while water is added from the tap so that the input of water exactly equals the outflow. In this idealized situation, the amount of water in the tub will remain at a constant level while there is a constant flow of water through the bathtub "system."
If the flow of water at either end of the system changes, the level in the tub will change at a rate that depends on the severity of the change.
In nature, the changes occur more or less randomly and may take place on any time scale. Over time, changes in one direction tend to be balanced by equal changes in the other direction.
Over geologic time, changes in one direction may dominate, causing permanent, drastic changes in climate or landscapes, and in evolutionary theory may lead to speciation.
Variations and fluctuations in nature that include sun, wind, tides, currents, rain, temperature and humidity inevitably alter the balance on one side or the other of the equation. In natural systems, when a change takes place an adjustment is made to compensate and bring the system back to equilibrium, even though a true equilibrium state is seldom reached and never maintained for long periods.
Dynamic equilibrium is a working model for many natural processes in the natural and human sciences, as the following examples will illustrate.
A state of chemical equilibrium is when a reversible reaction proceeds at equal rates in both directions such that the amount of reactants and products remain constant. Change the amount of any reactant or product, or ambient conditions such as temperature, however, and the rate of reaction in one direction or the other changes until a new dynamic equilibrium is reached, resulting in a different proportion of reactants and products.
Dynamic equilibrium applies within a covered jar partially filled with water. When first covered, the air in the jar is not saturated with water vapor. But evaporation soon saturates the air, at which point there is a balance between condensation and evaporation as equal number of molecules of water escape into the air and and return to the liquid. Cooling or warming the jar disrupts the equilibrium, causing an excess in one direction or the other until a new dynamic equilibrium is established between the liquid and the humidity of the air.
Another case of dynamic equilibrium is the salinity of the ocean. Salinity is maintained at a relatively constant level as dissolved solids are continually being added through input by streams, but are also being removed by various chemical, physical, or biological processes. The "residence time," or average time from input to output, is different for each constituent, such as sodium ions or magnesium ions, reflecting the balance between the input and output rates of the different constituents.
A river or stream seeks to maintain a dynamic equilibrium, but generally the conditions change too fast for equilibrium to be maintained for very long. Mostly this is due to the immense variability of rainfall within the river's drainage area, but the equilibrium may also be upset by a landslide that adds more sediment, or dams the river somewhere along its course. With every change in the amount of water or sediment, the stream adjusts the size of sediment that it carries or deposits in the stream bed. The result is shifting sandbars and changes in the course of the river as sediment moves downstream in a series of stops and starts to eventually reach the sea where it may become part of the beach system.
The beach is another example of a system in a state of dynamic equilibrium. The sand on a beach may seem to come and go in human time, but over geologic time, and without human intervention, there is a state of dynamic equilibrium. Any given grain of sand is in the beach system for a relatively short time in geologic terms. In a natural setting, there is a constant input to the beach system from both onshore and offshore, while there is a balanced and continual loss off material to the deeper offshore zone.
Intervention in the form of groins, seawalls, channelizing streams, or creating artificial harbors disrupts the movement of sand through the beach system and will ultimately cause changes elsewhere in the system as it attempts to regain equilibrium.
Oversteepening a hillside for construction often leaves the hillside unstable with the ultimate result being landslides that tend to bring the hillside back to a slope angle that is appropriate for the size and type of material.
Weathering of rocks is another example of dynamic equilibrium. For example, the minerals in volcanic rocks form at high temperatures. After cooling, the minerals are no longer in their equilibrium conditions and are thus unstable. The result is chemical weathering, during which atoms rearrange themselves, under the influence of water and oxygen, to form new minerals, clay and iron oxides, which are stable at earth surface temperatures and which form the bulk of our Hawaiian soils, the remainder having dissolved and washed into the sea.
Dynamic equilibrium is especially important to warm-blooded creatures such as ourselves. Homeostasis keeps our body temperature nearly constant. If we get too hot, the body initiates action to cool us; we sweat and the blood vessels near the skin's surface dilate to allow heat to escape. If we get too cold we shiver to generate metabolic heat and the capillaries contract to conserve the heat and divert blood away from the extremities to preserve the core temperature.
Equilibrium is important in the social sciences as well. In free market theory, the price of a product settles at an equilibrium price at which buyers can buy all they want and sellers can sell all they wish to sell. As the conditions of supply and demand change, the equilibrium price moves up or down in response.
Game theory in economics studies decision-making situations in which the choices of two or more individuals or groups influence one another. In game theory, the situations are called games and the decision makers are players.
The Nash equilibrium -- named for John Nash, the subject of the film "A Beautiful Mind" -- for which he won the Nobel prize, is an optimum strategy for all players in the game in which no one player benefits from changing strategy while all other players keep theirs the same.
Psychologically, we seek equilibrium between desire and satisfaction, in relationships between giving and taking, and between talking and listening. In reflective equilibrium, we find a state in which the consequences of one's general principles are consistent with one's opinions about individual cases and actions.
Much of the theoretical work in the various sciences has been done with equilibrium systems as an idealized way of understanding the processes. In the last half of the 20th century, though, theorists began to explore the dynamics of nonequilibrium systems.
Ilya Prigogine, a Russian-born Belgian theoretical chemist, was awarded the Nobel Prize for chemistry in 1977 for his contributions to the field of nonequilibrium thermodynamics. Subsequent studies of nonequilibrium systems has produced surprising results that relate to "chaos theory," a rapidly developing field of study that attempts to find underlying patterns in what appears to random variations, such as changes in the weather or the stock market.
One of the most interesting aspects of nonequilibrium dynamics is the finding that the further out of equilibrium a system is the more likely it is to form organized structures.
A good example is a bottle full of water. Sitting upright on the table it is in a state of stable equilibrium. The water is calm and completely homogeneous.
Tip the bottle upside down and the equilibrium state is disturbed. As water tries to escape from the inverted bottle and air tries to enter, organized structures of bubbles form within the bottle. A slight input of energy in the form of shaking the bottle will cause the water inside to swirl violently like a miniature upside-down tornado.
The swirling "tornado" is not only a highly organized structure, it is also the fastest and most efficient way of emptying the bottle.
Although the study of equilibrium dynamics has been fruitful in understanding many aspects of the physical and social world, the application of the new tools of chaos and nonequilibrium dynamics holds promise for increasing our understanding of many processes such as weather that were beyond the reach of the classical equilibrium studies.
Richard Brill picks up
where your high school science teacher left off. He is a professor of science
at Honolulu Community College, where he teaches earth and physical
science and investigates life and the universe.
He can be contacted by e-mail at
rickb@hcc.hawaii.edu