A 1-inch layer of air
keeps planes aloft
The Wright Brothers' initial flight, on Dec. 17, 1903, may turn out to have as great an impact on history as the control of fire, which even today powers all flight.
Flight has always been one of mankind's greatest dreams and most sought after technologies, and as great as their achievement was, it did not occur in an intellectual vacuum. They borrowed and learned from their predecessors' successes as well as their failures, but they introduced two major innovations that paved the way for modern aviation engineering. One was the wind tunnel and its associated culture of research. The other was the use of movable control surfaces. Previous glider flights had relied upon shifting body weight much like today's hang gliders, but the much heavier gliders did not respond quickly. Lest we forget the most successful glider and designer, from which the Wrights borrowed heavily, Otto Lilenthal died in a crash although he had successfully sailed up to 1,000 feet in previous flights.
It took just the right combination of power and control, and the Wright Brothers found it first, partly by accident but mostly by design.
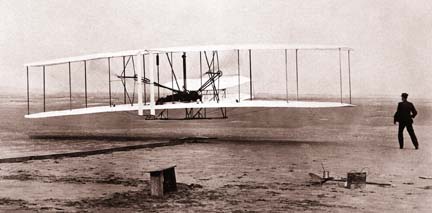
ASSOCIATED PRESS PHOTOS
Whether it is the Wright's first flight, above, or an aerobatic show, below, the same laws of physics prevail. A boundary layer of air about one inch thick wraps around the wing and is translated into lift.
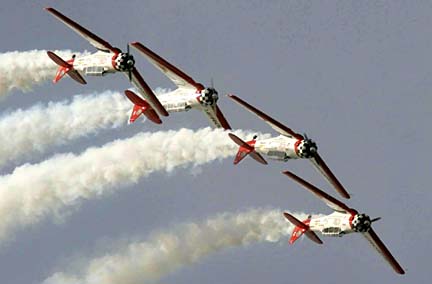
|
|
In order to fly, an airplane must generate enough engine power to create sufficient lifting force to overcome both the weight of the airplane and drag caused by air friction. The name, "airPLANE" is a misnomer and it is easy to see that there must be something else other than the planing force involved in lifting a heavier-than-air flying machine. A boat can plane on water at relatively low speeds, and it doesn't take a great deal of power to plane a sheet of plywood on water. But water is a hundred times more viscous than air, so it would take a speed 100 times greater and power 10,000 times greater to plane that same piece of plywood in air.
Airplanes flew and the aviation industry flourished long before anyone really understood what holds them up, mostly developed through trial and error in the wind tunnel and on the test range. The traditional answer to the question of the lifting force is the Bernoulli effect, named after the scientist who first described and explained it. Bernoulli described how increasing the speed of a fluid such as water or air lowers its pressure. Spray bottles and carburetors in cars (old cars, before there were fuel injectors) use the Bernoulli principle, and it works well for describing confined fluids in tubes and pipes, but not for unconfined air flowing over airplane wings.
Aeronautical engineers now understand that the Bernoulli effect provides some lift at low speeds, but the effect is small and insignificant in most aircraft. Likewise the planing force provides some lift, but both effects together still can not account for the lift of a working wing.
Computing power and concomitant software are important contributors to the science and technology of flight not only for their ability to quickly calculate and display vector fields in millions of colors in four dimensions. That alone is truly amazing, but the ability to design and test wing, fuselage and control surfaces in cyberspace cuts the time, the cost and the risk to human life to develop prototypes. As yet, cybermodels cannot completely replace physical models in a wind tunnel, just as the wind tunnel cannot completely mimic all the details of full-scale flight.
As with most technologies, the practical aspects of powered flight preceded the theoretical understanding of the principles behind it, but understanding developed rapidly in the latter part of the 20th century. Once the principles were known, technological growth accelerated, and continues to accelerate today as new aircraft are designed that have wing efficiencies far beyond the imaginations of early engineers. Nearly as unbelievable is the fact that the principles underlying flight are part of an ever-expanding world-view of physical consistency in the world of science. The laws that govern the lift of an airplane are all part of the same physics that ultimately determine the flight of a basketball toward the hoop, the motion of a Mars probe and the light that appears in the lamp when you flip the switch.
The explanation of the lift on a wing based on the Bernoulli principle is popular partly because it is easy to understand, and it makes sense, although it is incorrect. It says that air flowing over the top surface of the wing accelerates, thereby lowering the pressure above the wing. The problem is that the air above (and below) the wing is not confined like the air in a tube. Besides that, the explanation focuses solely on the shape of the wing and doesn't explain inverted flight. It also does not explain the dependence of lift on the angle of attack of the wing regardless of its shape, and such lesser known phenomena as the ground effect, power curves and high-speed stalls. The dynamic theory explains all of these things, and produces mathematical models that behave very close to the way actual wings behave .
Unfortunately for those of us who are not conversant with differential equations, the mathematical description that aeronautical engineers use requires this complex mathematics to calculate, and sophisticated computer software to simulate the lift of a wing. They are powerful tools for the engineer, but are neither intuitive nor accessible to most of us to help us to understand the forces that lift and keep aloft an airplane.
Calculations show that to generate enough lift to raise even a small airplane by the Bernoulli effect alone, the top of the curved wing would have to be one and a half times longer than that of the bottom. The shape of such a wing would be ridiculously "fat," and obviously not the rather thin shape of actual wings.
On the other hand, a small single-wing, single-engine aircraft would have to fly faster than 400 mph to generate lift by the Bernoulli principle alone using the wings that it flies with today at 80 mph.
There is nothing wrong with the Bernoulli principle. It is simply insufficient to explain flight. Bernoulli explained the principle using Newton's laws, and sometimes it is best to go back to the basics. We can do that, invoke Newton's Laws and use a little intuition to explain where the wing gets its lift.
Newton's laws are three simple statements that form the backbone of physics. They simply note that to change the direction or speed of anything a force must be exerted on it by something else, and that forces always occur in opposing pairs, one exerted on an object, the other exerted by the object. There is no such thing as a single, unopposed force just as a single hand cannot clap. As an example, a coffee cup sitting on a table exerts a force on the table (its weight) and the table puts an equal and opposite force on the cup to hold it up.
What really lifts the wing is that when a curved wing is tilted at an appropriate angle it pumps tremendous amounts of air downward, most of it from above the wing. The wing exerts a downward force on the air to deflect it. Consistent with Newton's laws, when the wing exerts a downward force on air, air exerts an upward force on the wing. Upward force on wing equals lift. That seems simple enough, but the surprise is the amount of air that is deflected and where it comes from.
Air doesn't have much mass, so the wing has to deflect a lot of air. For example, a typical small, single-engine airplane flying at 130 mph must generate around 2,300 pounds of lift. To do so it must pump downward as much as 5 tons of air per second. Imagine the amount of air deflected downward by an 800,000-pound Boeing 747 flying at 500 mph.
To divert such a huge quantity of air the wing must move air far above it as it scoops a hole through the air. Air from above the wing fills the void and pulls down on the air above it. The wing acts as a scoop, pulling air down from above it. Wing pulls air down, air pulls wing up.
An instantaneous snapshot of air movement around the wing as an aircraft passes by would actually show the air molecules moving in a circular loop above the wing, upward at the leading edge, downward at the trailing edge, extending upward far above the wing, with the greatest speed just above the highest part of the wing. Below the wing, the air is pushed forward, but only very slightly. This "bound vortex" model is the one that is applied mathematically with great success by engineers and computer modelers.
The key to understanding how the wing is able to affect and divert the air above it is viscosity. When a moving fluid, such as air or water, contacts a curved surface it will try to follow that surface. Try this at the kitchen sink. Run a thin stream of water so that it just touches the side of a drinking glass. The smaller the stream the better it works. Instead of flowing straight down, the water wraps around the glass. From Newton's laws, we know that for the fluid to bend there must be a force acting on it, and that the fluid must put an equal and opposite force on the object that caused it to bend. In this case it's the glass, but on an aircraft it's the wing.
The viscosity of air is very small, but it is enough for the air molecules to want to stick to the surface, however slightly. That's why there is dust even on the downwind side of fan blades, and why it is impossible to blow dust off a smooth surface. The thin band of air around the wing that is partially stuck to the wing is called the "boundary layer." The boundary layer is only about an inch thick on a 747, but it's thick enough. Just above the boundary layer, the air is moving every so slightly. The farther from the surface the faster it is moving. As the air near the surface of the wing changes its velocity, it is bent toward the surface. The force required to wrap air around the wing is translated into lift. As long as the curved wing is not too curved, the air will follow its surface. This is the same effect that causes a baseball to curve and which gives loft to a dimpled golf ball.
In actual flight, the pilot adjusts the angle of attack of the wing to compensate the lift for the speed and load. Typically, the lift increases up to an angle of attack of about 15 degrees and falls off rapidly at greater angles. This angle maximizes the ability of the air to remain in the viscous boundary layer, which happens just before the air above it breaks into turbulence. Beyond that, the air separates from the wing, and the wing stalls. Increased angle of attack also causes increased drag, which must be compensated for at the expense of power to maintain airspeed.
The dynamics of flight are complex, and the current theories are fruitful, but the fundamentals behind them are the same laws of physics, the effects of which we encounter in daily life when we hit a golf ball or drive a car. That's why we call them laws. That we have understood and exploited them to fly amazes me whenever I'm at 35,000 feet flying at near the speed of sound. But I also think about how that one-inch thick layer of air keeps 400 people and the half-a-million-pound flying locomotive aloft.
Richard Brill picks up
where your high school science teacher left off. He is a professor of science
at Honolulu Community College, where he teaches earth and physical
science and investigates life and the universe.
He can be contacted by e-mail at
rickb@hcc.hawaii.edu